Foundation Topics
Introduction to Routing Protocols
Many IP routing protocols exist, in part due to the long history of IP; however, if you compare all the IP routing protocols, they all have some core features in common. Each routing protocol causes routers (and Layer 3 switches) to
- Learn routing information about IP subnets from other neighboring routers
- Advertise routing information about IP subnets to other neighboring routers
- Choose the best route among multiple possible routes to reach one subnet, based on that routing protocol’s concept of a metric
- React and converge to use a new choice of best route for each destination subnet when the network topology changes—for example, when a link fails
All the routing protocols discussed in this chapter do these same four functions, but the protocols differ in other ways. The rest of this chapter works through enough of the logic and features of each routing protocol so that you can see the differences, while understanding the basics of how each routing protocol learns routes, advertises routes, picks the best route, and converges when the network changes.
History of Interior Gateway Protocols
Historically speaking, RIP Version 1 (RIPv1) was the first popularly used IP routing protocol, with the Cisco-proprietary Interior Gateway Routing Protocol (IGRP) being introduced a little later, as shown in Figure 20-1.
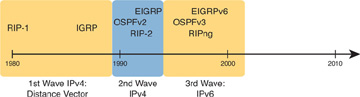
Figure 20-1 Timeline for IP IGPs
By the early 1990s, business and technical factors pushed the IPv4 world toward a second wave of better routing protocols. RIPv1 and IGRP had some technical limitations, even though they were great options for the technology levels of the 1980s. The huge movement toward TCP/IP in the 1990s drove the need for better IPv4 routing protocols. In the 1990s, many enterprises migrated from older vendor-proprietary networks to networks built with routers, LANs, and TCP/IP. These businesses needed better performance from their routing protocols, including better metrics and better convergence. All these factors led to the introduction of a new wave of IPv4 Interior routing protocols: RIP Version 2 (RIPv2), OSPF Version 2 (OSPFv2), and EIGRP.
Comparing IGPs
What is an IGP in the first place? All the routing protocols mentioned so far in this chapter happen to be categorized as Interior Gateway Protocols (IGPs) rather than as Exterior Gateway Protocols (EGPs). First, the term gateway was used instead of router in the early days of IP routing, so the terms IGP and EGP really do refer to routing protocols. The designers of some routing protocols intended the routing protocol for use inside one company or organization (IGP), with other routing protocols intended for use between companies and between Internet service providers (ISPs) in the Internet (EGPs).
This chapter falls back to using the term IGP when talking about all the routing protocols mentioned in this chapter.
When deploying a new network, the network engineer can choose between a variety of IGPs. Today, most enterprises use EIGRP and OSPFv2. RIPv2 has fallen away as a serious competitor, in part due to its less robust hop-count metric, and in part due to its slower (worse) convergence time. This chapter discusses enough of the basics of all of these IGPs so that you get a sense of some of the basic trade-offs when comparing these routing protocols. A few key comparison points are as follows:
- The underlying routing protocol algorithm: Specifically, whether the routing protocol used logic referenced as distance vector (DV) or link state (LS).
- The usefulness of the metric: The routing protocol chooses which route is best based on its metric; so the better the metric, the better the choices made by that routing protocol.
- The speed of convergence: How long does it take all the routers to learn about a change in the network and update their IPv4 routing tables? That concept, called convergence time, varies depending on the routing protocol.
- Whether the protocol is a public standard or a vendor-proprietary function: RIP and OSPF happen to be standards, defined by RFCs. EIGRP happens to be defined by Cisco, and until 2013, was kept private.
For example, RIP uses a basic metric of hop count. Hop count treats each router as a hop, so the hop count is the number of other routers between a router and some remote subnet. RIP’s hop-count metric means that RIP picks the route with the smallest number of links and routers. However, that shortest route may have the slowest links; a routing protocol that uses a metric based in part on link speed (called bandwidth) might make a better choice. In contrast, EIGRP’s metric calculation uses a math formula that gives routes with slow links a worse metric, and routes with fast links a lower metric, so EIGRP prefers faster routes.
For example, Figure 20-2 shows two copies of the same topology. The topology shows three Nexus switches configured to act as Layer 3 switches. The figure focuses on router B’s route to a subnet off router A. As you can see on the left in the figure, RIP on router B chooses the shorter hop route over the top of the network, over the single link, even though that link runs at 1 Gbps. EIGRP, on the right side of the figure, chooses the route that happens to have more links through the network, but both links have a faster bandwidth of 10 Gbps.
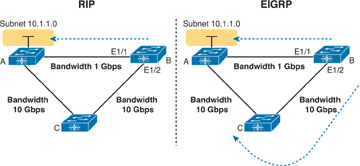
Figure 20-2 EIGRP Choosing the Longer but Better Route to Subnet 10.1.1.0
On another comparison point, the biggest negative about EIGRP has traditionally been that it required Cisco routers. That is, using EIGRP locked you into using Cisco products, because Cisco kept EIGRP as a Cisco proprietary protocol. In an interesting change, Cisco published EIGRP as an informational RFC in 2013, meaning that now other vendors can choose to implement EIGRP as well. In the past, many companies chose to use OSPF rather than EIGRP to give themselves options for what router vendor to use for future router hardware purchases. In the future, it might be that you can buy some routers from Cisco, some from other vendors, and still run EIGRP on all routers.
For reference and study, Table 20-2 lists several features of OSPFv2 and EIGRP, as well as RIPv2. Note that the table includes a few features that have not yet been introduced (but will be introduced before the end of the chapter).
Table 20-2 Interior IP Routing Protocols Compared
Feature |
RIPv1 |
RIPv2 |
EIGRP |
OSPF |
Distance vector (DV) or link state (LS) |
DV |
DV |
DV 1 |
LS |
Default metrics based on link bandwidth |
No |
No |
Yes |
Yes |
Convergence time |
Slow |
Slow |
Fast |
Fast |
Originally Cisco proprietary |
No |
No |
Yes |
No |
Uses areas for design |
No |
No |
No |
Yes |
Routing updates are sent to a multicast IP address |
No |
Yes |
Yes |
Yes |
Classless/supports VLSM |
No |
Yes |
Yes |
Yes |
Distance Vector Basics
Each IGP can be categorized based on its internal logic, either DV or LS. As a starting point to better understand IGPs, the next few pages explain more about how a DV protocol actually exchanges routing information. These pages use RIP as an example, showing RIP’s simple hop-count metric, which, although a poor option in real networks today, is a much simpler option for learning.
The Concept of a Distance and a Vector
The term distance vector describes what a router knows about each route. At the end of the process, when a router learns about a route to a subnet, all the router knows is some measurement of distance (the metric) and the next-hop router and outgoing interface to use for that route (a vector, or direction).
Figure 20-3 shows a view of both the vector and the distance as learned with RIP. The figure shows the flow of RIP messages that cause R1 to learn some IPv4 routes, specifically three routes to reach subnet X:
- The four-hop route through R2
- The three-hop route through R5
The two-hop route through R7
Figure 20-3 Information Learned Using DV Protocols
DV protocols learn two pieces of information about a possible route to reach a subnet:
- The distance (metric)
- The vector (the next-hop router)
In Figure 20-3, R1 learns three routes to reach subnet X, through three different neighboring routers. If R1 had learned only one route to subnet X, R1 would use that route. However, having learned three routes to subnet X, R1 picks the two-hop route through next-hop router R7 because that route has the lowest RIP metric.
While Figure 20-3 shows how R1 learns the routes with RIP updates, Figure 20-4 gives a better view into R1’s DV logic. The figure shows R1’s three competing routes to subnet X as vectors, with longer vectors for routes with larger metrics. R1 knows three routes, each with
- Distance: The metric for a possible route
- Vector: The direction, based on the next-hop router for a possible route
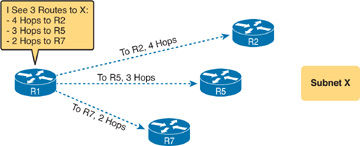
figure 20-4 Graphical Representation of the DV Concept
Full Update Messages and Split Horizon
Some DV protocols, such as RIP (both RIPv1 and RIPv2), send periodic full routing updates based on a relatively short timer. Specifically, full update means that a router advertises all its routes, using one or more RIP update messages, no matter whether the route has changed or not. So, if a route does not change for months, the router keeps advertising that same route over and over.
Figure 20-5 illustrates this concept in an internetwork with two Nexus switches configured as Layer 3 switches, with four total subnets. The figure shows both routers’ full routing tables, and lists the periodic full updates sent by each router.
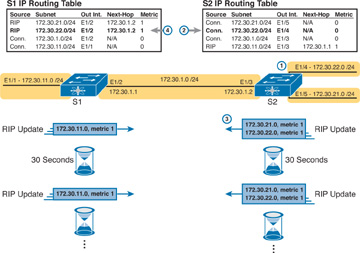
Figure 20-5 Normal Steady-State RIP Operations: Full Update with Split Horizon
This figure shows a lot of information, so take the time to work through the details. For example, consider what switch S1 learns for subnet 172.30.22.0/24, which is the subnet connected to S2’s E1/4 interface:
- S2 interface E1/4 has an IP address, and is in an up/up state.
- S2 adds a connected route for 172.30.22.0/24, off interface E1/4, to R2’s routing table.
- S2 advertises its route for 172.30.22.0/24 to S1, with metric 1, meaning that S1’s metric to reach this subnet will be metric 1 (hop count 1).
- S1 adds a route for subnet 172.30.22.0/24, listing it as a RIP learned route with metric 1.
Also, take a moment to focus more on the route learned at Step 4: The bold route in S1’s routing table. This route is for 172.30.22.0/24, as learned from S2. It lists S1’s local E1/2 interface as the outgoing interface because S1 receives the update on that interface. It also lists S2’s IP address of 172.30.1.2 as next-hop router because that’s the IP address from which S1 learned the route.
Monitoring Neighbor State with Periodic RIP Updates
RIPv1 and RIPv2 also send periodic updates, as shown in the bottom of Figure 20-5. That means that each router sends a new update (a full update) on a relatively short time period (30 seconds with RIP).
Many of the early DV protocols used this short periodic timer, repeating their full updates, as a way to let each router know whether a neighbor had failed. Routers need to react when a neighboring router fails or if the link between two routers fails. If both routers on a link must send updates every 30 seconds, when a local router no longer receives those updates, it knows that a problem has occurred, and it can react to converge to use alternate routes.
Note that newer DV protocols, such as EIGRP, do not require routers to keep sending updates for the purpose of tracking the state of the neighbor. Instead, they both define a simple hello protocol that allows the routers to send short messages to each other, instead of the long full routing updates, for the purpose of knowing when a neighbor fails.
Split Horizon
Figure 20-5 also shows a common DV feature called split horizon. Note that both routers list all four subnets in their IP routing tables. However, the RIP update messages do not list four subnets. The reason? Split horizon.
Split horizon is a DV feature that tells a router to omit some routes from an update sent out an interface. Which routes are omitted from an update sent out interface X? The routes that would like interface X as the outgoing interface. Those routes that are not advertised on an interface usually include the routes learned in routing updates received on that interface.
Split horizon is difficult to learn by reading words, and much easier to learn by seeing an example. Figure 20-6 continues the same example as Figure 20-5, but focusing on S1’s RIP update sent out S1’s E1/2 interface to S2. Figure 20-6 shows S1’s routing table with three light-colored routes, all of which list E1/2 as the outgoing interface. When building the RIP update to send out E1/2, split-horizon rules tell S1 to ignore those light-colored routes. Only the bold route, which does not list E1/2 as an outgoing interface, can be included in the RIP update sent out E1/2.
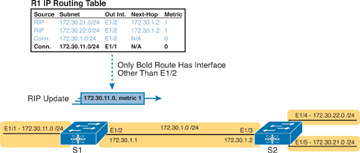
Figure 20-6 R1 Does Not Advertise Three Routes Due to Split Horizon
Route Poisoning
DV protocols help prevent routing loops by ensuring that every router learns that the route has failed, through every means possible, as quickly as possible. One of these features, route poisoning, helps all routers know for sure that a route has failed.
Route poisoning refers to the practice of advertising a failed route, but with a special metric value called infinity. Routers consider routes advertised with an infinite metric to have failed.
Figure 20-7 shows an example of route poisoning with RIP, with S2’s E1/4 interface failing, meaning that S2’s route for 172.30.22.0/24 has failed. RIP defines infinity as 16.
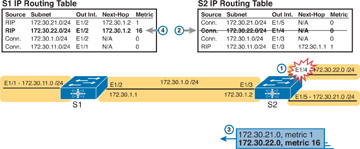
Figure 20-7 Route Poisoning
Figure 20-7 shows the following process:
- S2’s E1/4 interface fails.
- S2 removes its connected route for 172.30.22.0/24 from its routing table.
- S2 advertises 172.30.22.0 with an infinite metric (which for RIP is 16).
- Depending on other conditions, S1 either immediately removes the route to 172.30.22.0 from its routing table, or marks the route as unusable (with an infinite metric) for a few minutes before removing the route.
By the end of this process, router S1 knows for sure that its old route for subnet 172.30.22.0/24 has failed, which helps S1 avoid introducing looping IP routes.
Each routing protocol has its own definition of an infinite metric. RIP uses 16, as shown in the figure, with 15 being a valid metric for a usable route. EIGRP has long used 232 – 1 as infinity (a little more than 4 billion), with some Cisco products bumping that value to 256 – 1 (more than 1016). OSPFv2 uses 224 – 1 as infinity.
The previous few pages focused on DV concepts, using RIP as an example. This chapter next turns the focus to the particulars of both RIPv1 and RIPv2.