- 1.1 The Frontier: Millimeter Wave Wireless
- 1.2 A Preview of MmWave Implementation Challenges
- 1.3 Emerging Applications of MmWave Communications
- 1.4 Contributions of This Textbook
- 1.5 Outline of This Textbook
- 1.6 Symbols and Common Definitions
- 1.7 Chapter Summary
1.2 A Preview of MmWave Implementation Challenges
Implementation challenges for mmWave communication involve many layers of the communications stack. At the hardware level of the PHY, antennas are a major challenge. To minimize costs, mmWave chipset vendors may prefer to exploit the short carrier wavelength by incorporating antennas or antenna arrays directly on-chip or in-package. For the simplest and lowest-cost solutions, high-gain single chip solutions are attractive [RGAA09]. Single-antenna solutions, however, must overcome the challenges of low on-chip efficiencies whereas in-package antennas must overcome lossy package interconnects. MmWave systems may also employ many closely spaced antennas in packages or on circuit boards that are much smaller than a centimeter when using high-permittivity materials. Adaptive or switched-beam antenna arrays can provide transmit and receive antenna gain, but require protocol modifications at the signal processing level of the PHY and the data link layer to direct the beams.
A cornerstone of low-cost mmWave circuits is the use of CMOS or SiGe technology. Silicon on Insulator (SOI) CMOS processes are also attractive for high-end applications as they provide impressive quality (Q) factors due to reduced values for parasitic capacitances and inductances. SOI processes, however, suffer from increased costs compared with standard CMOS with a joined device channel and substrate. Because CMOS processes have now reached transit frequencies of hundreds of gigahertz, single-chip mmWave systems are feasible, complete with a digital baseband and mmWave analog front end. On-chip integration will also facilitate techniques like mixed-signal equalization [TKJ+12] [HRA10] that may improve the performance of complete systems versus multichip solutions. Unfortunately, foundries do not yet report relative permittivities or loss-tangents for process materials at mmWave frequencies in process design kits (PDKs), forcing early developers to measure these critical parameters until they are provided.
Communications signal processing at mmWave is also met with new challenges. Although mmWave wireless links can be modeled using conventional linear complex baseband system theory, the characteristics of mmWave wireless propagation combined with mmWave hardware design requirements produce unique design decisions at the PHY. Modulation and equalization algorithm selection must take into account the derived tradeoff between beam steering complexity and equalization complexity. For example, a mmWave system that uses omni directional antennas can suffer from severe ISI due to the multipath channels that cause successive symbols arriving at a receiver to overlap and interfere with each other [Rap02]. Fig. 1.13 illustrates the temporal and spatial variations of a typical omnidirectional 60 GHz impulse response and shows how multipath components may induce tens or even hundreds of nanoseconds of delay. The channel shown in Fig. 1.13 has a delay spread of 65.9 ns, which could potentially spread a 60 GHz signal over tens to hundreds of symbol periods (e.g., this much spread would smear a signal over 120 symbols in the Single Carrier-Physical Layer of the IEEE 802.15.3c standard) [DMRH10]. A device operating in this environment would either need nonlinear equalization algorithms in the PHY and/or very long equalization periods, both of which would increase the complexity of the device (possibly erasing digital complexity benefits of 60 GHz relative to lower frequency systems). Directional beam steering antennas such as antenna arrays may be used to reduce the RMS delay spread seen by the device, but beam steering also results in an additional computational burden.
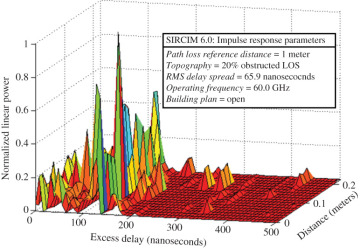
Figure 1.13 Long delay spreads characterize wideband 60 GHz channels and may result in severe inter-symbol interference, unless directional beamforming is employed. Plot generated with Simulation of Indoor Radio Channel Impulse Response Models with Impulse Noise (SIRCIM) 6.0 [from [DMRH10]© IEEE].
Above the PHY, the medium access control (MAC) of mmWave devices must also consider unique design factors. Most of the computational burden of beam steering would fall in the MAC layer. In addition to reducing complexity by optimal co-design of beam-steering and modulation algorithms, beam steering presents problems related to neighbor discovery and hidden and exposed nodes in a network. Neighbor discovery, which refers to the link protocol that manages link activation and maintenance, is especially difficult with beam steering and mobile devices. The hidden node problem, in which a coordinating device is unable to prevent an interfering device from transmitting, is challenging enough in microwave systems with omnidirectional antennas. The addition of very directional mmWave antennas (to combat mmWave path loss) only compounds this problem. Exposed nodes, which are prevented from communicating due to interference, are more likely to occur with conventional MAC protocols at mmWave because of the directionality of “all clear” messages with mmWave antennas [DMRH10].