- 1.1 Electromotive Force (emf)
- 1.2 Electrical Power
- 1.3 Power Delivery
- 1.4 Power Integrity (PI)
- 1.5 Exercises
- References
1.2 Electrical Power
Electrical power is defined as the product of voltage and current, where the latter is the rate of flow of electric charges, represented by I or i. The SI unit of current is the ampere. Instantaneous power, therefore, is the product of instantaneous voltage and instantaneous current, or:
Equation 1-9

Instantaneous power Pi is often averaged over a representative time duration to yield average power. In alternating current systems with sinusoidally varying potentials, instantaneous voltages and currents are often vectors with a phase angle difference, leading to:
Equation 1-10

where V and I are amplitudes or peak absolute values for voltage and current, ω is the vector angular velocity, and φ is the phase difference between voltage and current vectors. Solving further using known trigonometric relationships [3], we find:
Equation 1-11

which, when averaged over one or more cycles, given that the average of any sinusoidal function over its period is zero, yields:
Equation 1-12

which may also be written as:
Equation 1-13
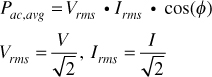
where Vrms and Irms are the root-mean-square or “effective” values of voltage and current, respectively. The cosine of the phase angle between the alternating voltage and alternating current is also called the power factor, or the factor by which the product of effective voltage and current must be multiplied to yield true alternating current (AC) power. For current flow that is 90 degrees or one-quarter cycle out of phase with voltage, the power factor is 0.
Multiplication of the current vector with the power factor yields the component of the current vector in phase with the voltage vector: It is only this component that results in power delivered or consumed. Orthogonal relationships between voltage and current vectors, which exist in purely reactive components such as ideal capacitors and inductors, therefore do not result in power consumed, though large currents may flow through such components in response to applied alternating or otherwise varying voltages.
1.2.1 Physical Analogy for Power
Electrical power is the product of voltage and current, where the latter is charge transferred over time. Following the force-voltage analogy in our earlier discussion in this chapter, power should be equivalent to force applied for a distance over time, or a velocity (rate) at which force is applied and work done. This provides an intuitive definition for power as the rate at which energy is absorbed or produced. The greater the power, the greater the capacity to provide energy, and the greater the rate at which energy is absorbed or consumed.
The SI unit of power is the watt, with the symbol W. If 1 joule of work is done in 1 second, the power consumed is 1 watt. Following this definition, and from the earlier relationship between voltage, work, and charge:
Equation 1-14

By the same definition, energy, or work done, is the product of power and time. Higher power consumption therefore leads to higher energy absorption in a unit of time. This is demonstrated physically in the heating of materials. Higher power directly translates to faster increases in temperature and greater heat buildup.
1.2.2 Sources of Electrical Power
Electrical energy and power can be generated in numerous ways. Natural electrical energy is generated through static electricity, or the physical separation and transport of charges, resulting in lightning and the commonly observed crackles in everyday objects that rub against each other. Static electricity has been employed in Van de Graaf generators [2] to produce more than a million volts.
The most common method of electricity generation is electromagnetic induction, where mechanical energy is employed to turn an electrical generator. Sources of mechanical energy include nuclear energy, solar thermal energy, the flow of water, wind, ocean tides, and even ocean waves. The other common method of electrical energy generation is through electrochemistry, or a direct conversion of chemical energy into electrical energy, as seen in electric batteries and fuel cells. Photovoltaic conversion, the transformation of energy in sunlight directly into electrical energy, is now widely employed as well. Supercapacitors, with extremely high energy storage density, often replace or augment electric batteries. Other forms of electricity generation are thermo-electricity, a direct transformation of heat into emf in thermocouples, and piezo-electricity, a transformation of mechanical strain in crystals into electricity. These energy conversion devices are employed primarily as sensors, though some are used in energy harvesting, a process in which energy is extracted from the ambient environment to provide electrical power to small, autonomous devices. Radio frequency (RF) fields are also now used to harvest small amounts of energy.
1.2.3 Powering Electrical and Electronic Circuits and Systems
All electrical and electronic systems function using electrical energy. These systems now exist in tremendous variety, assisting human activity in every manner imaginable. They employ electrical energy to perform useful work, transforming energy into other forms, such as motion (kinetic energy), light (visible electromagnetic energy), and heat (thermodynamic energy). They may also alter quantities of electrical energy, producing higher or lower potential differences and periodically varying potential differences.
Different systems are designed to employ different forms of electrical energy. Most large machines are designed to function using the alternating current (AC) form of electrical energy. Due primarily to its ease of transformation and transmission, AC was deemed most suitable for the transmission of electrical energy from generating stations to distributing stations and end users. Traditional lighting systems, such as incandescent lamps and fluorescent lamps, are designed to operate using AC electrical power. Many household machines are also designed to run using AC voltages reduced to a relatively safe level. Such AC electrical power employs a frequency of 50, 60, or 440 cycles per second, and an amplitude from 110 V to 240 V or higher. AC power is often provided with multiple phases, or alternating voltages shifted in phase angle from each other, typically in three phases 120 degrees apart. Systems employing AC power are generally called “electrical” systems.
Electronic systems typically operate with direct current (DC, previously called galvanic current) electrical power. DC electrical power is characterized by a constant voltage (or potential difference supplied) and a unidirectional flow of electric charge. Most such systems operate at low voltage values that are one to two orders of magnitude below amplitudes common in AC electrical power. AC electrical power is readily convertible into DC through rectifiers, DC-to-DC converters, and filters. Nowadays, DC can also be readily converted into AC through electronic systems called inverters. High-voltage direct current is sometimes employed to transmit large amounts of power from remote generation sites or to interconnect AC power grids. DC power is also finding greater use in energy-efficient solid-state lighting.2
Our discussion in succeeding sections and chapters will focus on direct current electrical power as the predominant form of electrical power energizing electronic systems, with specific reference to integrated circuits and systems.