Enzymes
Enzymes are usually proteins of high molecular weight (15,000 < MW < several million daltons) that act as catalysts. Recently, it has been shown that some RNA molecules are also catalytic, but the vast majority of cellular reactions are mediated by protein catalysts. RNA molecules that have catalytic properties are called ribozymes. Both ribozymes and enzymes are specific, versatile, and very effective biological catalysts, resulting in much higher reaction rates as compared to chemically catalyzed reactions under ambient conditions.
More than 2000 enzymes are known. Enzymes are named by adding the suffix -ase to the end of the substrate, such as urease, or to the end of the reaction catalyzed, such as alcohol dehydrogenase. Some enzymes have a simple structure, such as a folded polypeptide chain (typical of most hydrolytic enzymes). Many enzymes have more than one subunit. Some protein enzymes require a nonprotein group for their activity. This group is either a cofactor, such as metal ions, Mg, Zn, Mn, Fe, or a coenzyme, such as a complex organic molecule, nicotinamide adenine dinucleotide (NAD), flavin adenine dinucleotide (FAD), coenzyme A (CoA), or some vitamins. An enzyme containing a nonprotein group is called a holoenzyme. The protein part of this enzyme is the apoenzyme (holoenzyme = apoenzyme + cofactor). Enzymes that occur in several different molecular forms, but catalyze the same reaction, are called isozymes. Some enzymes are grouped to form enzyme complexes. Enzymes are substrate specific and are classified according to the reaction they catalyze. Major classes of enzymes and their functions are listed in Table 3.1.
Table 3.1. International Classification of Enzymes
Class No. |
Class Name |
Type of Reaction Catalyzed |
1 |
Oxidoreductases |
Transfer of electrons (hydride ions or H atoms) |
2 |
Transferases |
Group transfer reactions |
3 |
Hydrolases |
Hydrolysis reactions (transfer of functional groups to water) |
4 |
Lyases |
Cleavage of C—C, C—O, C—N, or other bonds by elimination, leaving double bonds or rings, or addition of groups to double bonds |
5 |
Isomerases |
Transfer of groups within molecules to yield isomeric forms |
6 |
Ligases |
Formation of C—C, C—S, C—O, and C—N bonds by condensation reactions coupled to cleavage of ATP or similar cofactor |
From Lehninger, Principles of Biochemistry, 6th ed., by David L. Nelson et al., Copyright 2013. All rights reserved. Reprinted by permission of W. H. Freeman
3.1. How Enzymes Work
Enzymes lower the activation energy of the reaction catalyzed by binding the substrate and forming an enzyme–substrate complex. Enzymes do not affect the free-energy change or the equilibrium constant. Figure 3.1 illustrates the action of an enzyme from the activation-energy point of view. For example, the activation energy for the decomposition of hydrogen peroxide varies depending on the type of catalysis. The activation energy of the uncatalyzed reaction at 20°C is 18 kilocalories per mole (kcal/mol), whereas the ∆E values for chemically catalyzed (by colloidal platinum) and enzymatically catalyzed (catalase) decomposition are 13 and 7 kcal/mol, respectively. That is, catalase accelerates the rate of reaction by a factor of about 108. This large change in rate for a relatively small change in activation energy is due to the exponential dependence of rate on activation energy.

Figure 3.1. Activation energies of enzymatically catalyzed and uncatalyzed reactions. Note that |ΔG°A2| < |ΔG°A1|.
The molecular aspects of enzyme–substrate interaction are not yet fully understood. This interaction varies from one enzyme–substrate complex to another. Various studies using x-ray and Raman spectroscopy have revealed the presence of the enzyme–substrate complex. The interaction between the enzyme and its substrate is usually by weak forces. In most cases, van der Waals forces and hydrogen bonding are responsible for the formation of enzyme–substrate complexes. The substrate binds to a specific site on the enzyme known as the active site. The substrate is a relatively small molecule and fits into a certain region on the enzyme molecule, which is a much larger molecule. The simplest model describing this interaction is the lock-and-key model, in which the enzyme represents the lock and the substrate represents the key, as described in Figure 3.2. The induced fit model is a more widely accepted mechanism for enzyme–substrate interaction where the active site of the enzyme goes through some conformational changes to bind the substrate. Enzymatic reaction takes place as a result of those conformational changes.
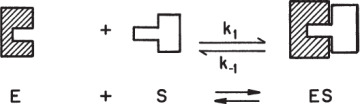
Figure 3.2. Schematic of the lock-and-key model of enzyme catalysis.
In multisubstrate enzyme–catalyzed reactions, enzymes can hold substrates such that reactive regions of substrates are close to each other and to the enzyme’s active site, which is known as the proximity effect. Also, enzymes may hold the substrates at certain positions and angles to improve the reaction rate, which is known as the orientation effect. In some enzymes, the formation of an enzyme–substrate complex causes slight changes in the three-dimensional shape of the enzyme. This induced fit of the substrate to the enzyme molecule may contribute to the catalytic activity of the enzyme, too. The enzymes lysozyme and carboxypeptidase A have been observed to change their three-dimensional structure upon complexing with the substrate. Enzyme catalysis is affected not only by the primary structure of enzymes but also by the secondary, tertiary, and quaternary structures. The properties of the active site of enzymes and the folding characteristics have a profound effect on the catalytic activity of enzymes. Certain enzymes require coenzymes and cofactors for proper functioning. Table 3.2 lists some enzymes and their cofactors and coenzymes.
Table 3.2. Cofactors (Metal Ions) and Coenzymes of Some Enzymes
Some Inorganic Ions That Serve as Cofactors for Enzymes
Ions |
Enzymes |
Cu2+ |
Cytochrome oxidase |
Fe2+ or Fe3+ |
Cytochrome oxidase, catalase, peroxidase |
K+ |
Pyruvate kinase |
Mg2+ |
Hexokinase, glucose 6-phosphatase, pyruvate kinase |
Mn2+ |
Arginase, ribonucleotide reductase |
Mo |
Dinitrogenase |
Ni2+ |
Urease |
Zn2+ |
Carbonic anhydrase, alcohol dehydrogenase, carboxypeptidase A and B |
Some Coenzymes That Serve as Transient Carriers of Specific Atoms or Functional Groups
Coenzyme |
Examples of Chemical Groups Transferred |
Dietary Precursor in Mammals |
Biocytin |
CO2 |
Biotin |
Coenzyme A |
Acyl groups |
Pantothenic acid and other compounds |
5'-Deoxyadenosylcobalamin (coenzyme B12) |
H atoms and alkyl groups |
Vitamin B12 |
Flavin adenine dinucleotide |
Electrons |
Riboflavin (vitamin B2) |
Lipoate |
Electrons and acyl groups |
Not required in diet |
Nicotinamide adenine dinucleotide |
Hydride ion (:H-) |
Nicotinic acid (niacin) |
Pyridoxal phosphate |
Amino groups |
Pyridoxine (vitamin B6) |
Tetrahydrofolate |
One-carbon groups |
Folate |
Thiamine pyrophosphate |
Aldehydes |
Thiamine (vitamin B1) |
From Lehninger, Principles of Biochemistry, 6th ed., by David L. Nelson et al., Copyright 2013. All rights reserved. Reprinted by permission of W. H. Freeman.