- 1.1 Introduction to Petroleum Reservoirs
- 1.2 History of Reservoir Engineering
- 1.3 Introduction to Terminology
- 1.4 Reservoir Types Defined with Reference to Phase Diagrams
- 1.5 Production from Petroleum Reservoirs
- 1.6 Peak Oil
- Problems
1.4 Reservoir Types Defined with Reference to Phase Diagrams
From a technical point of view, the various types of reservoirs can be defined by the location of the initial reservoir temperature and pressure with respect to the two-phase (gas and liquid) envelope as commonly shown on pressure-temperature (PT) phase diagrams. Figure 1.4 is the PT phase diagram for a particular reservoir fluid. The area enclosed by the bubble-point and dew-point curves represents pressure and temperature combinations for which both gas and liquid phases exist. The curves within the two-phase envelope show the percentage of the total hydrocarbon volume that is liquid for any temperature and pressure. At pressure and temperature points located above the bubble-point curve, the hydrocarbon mixture will be a liquid phase. At pressure and temperature points located above or to the right of the dew-point curve, the hydrocarbon mixture will be a gas phase. The critical point, where bubble-point, dew-point, and constant quality curves meet, represents a mathematical discontinuity, and phase behavior near this point is difficult to define. Initially, each hydrocarbon accumulation will have its own phase diagram, which depends only on the composition of the accumulation.
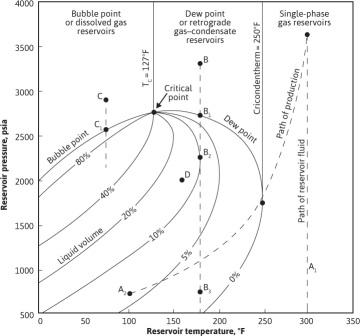
Figure 1.4 Pressure-temperature phase diagram of a reservoir fluid.
Consider a reservoir containing the fluid of Fig. 1.4 initially at 300°F and 3700 psia, point A. Since this point lies outside the two-phase region and to the right of the critical point, the fluid is originally in a one-phase gas state. Since the fluid remaining in the reservoir during production remains at 300°F, it is evident that it will remain in the single-phase or gaseous state as the pressure declines along path . Furthermore, the composition of the produced well fluids will not change as the reservoir is depleted. This is true for any accumulation with this hydrocarbon composition where the reservoir temperature exceeds the cricondentherm, or maximum two-phase temperature (250°F for the present example). Although the fluid left in the reservoir remains in one phase, the fluid produced through the wellbore and into surface separators, although the same composition, may enter the two-phase region owing to the temperature decline, as along line
. This accounts for the production of condensate liquid at the surface from a single-phase gas phase in the reservoir. Of course, if the cricondentherm of a fluid is below approximately 50°F, then only gas will exist on the surface at usual ambient temperatures, and the production will be called dry gas. Nevertheless, even dry gas may contain valuable liquid fractions that can be removed by low-temperature separation.
Next, consider a reservoir containing the same fluid of Fig. 1.4 but at a temperature of 180°F and an initial pressure of 3300 psia, point B. Here the fluid is also initially in the one-phase gas state, because the reservoir temperature exceeds the critical-point temperature. As pressure declines due to production, the composition of the produced fluid will be the same as reservoir A and will remain constant until the dew-point pressure is reached at 2700 psia, point B1. Below this pressure, a liquid condenses out of the reservoir fluid as a fog or dew. This type of reservoir is commonly called a dew-point or a gas-condensate reservoir. This condensation leaves the gas phase with a lower liquid content. The condensed liquid remains immobile at low concentrations. Thus the gas produced at the surface will have a lower liquid content, and the producing gas-oil ratio therefore rises. This process of retrograde condensation continues until a point of maximum liquid volume is reached, 10% at 2250 psia, point B2. The term retrograde is used because generally vaporization, rather than condensation, occurs during isothermal expansion. After the dew point is reached, because the composition of the produced fluid changes, the composition of the remaining reservoir fluid also changes, and the phase envelope begins to shift. The phase diagram of Fig. 1.4 represents one and only one hydrocarbon mixture. Unfortunately, this shift is toward the right and further aggravates the retrograde liquid loss within the pores of the reservoir rock.
Neglecting for the moment this shift in the phase diagram, for qualitative purposes, vaporization of the retrograde liquid occurs from B2 to the abandonment pressure B3. This revaporization aids liquid recovery and may be evidenced by decreasing gas-oil ratios on the surface. The overall retrograde loss will evidently be greater (1) for lower reservoir temperatures, (2) for higher abandonment pressures, and (3) for greater shift of the phase diagram to the right—the latter being a property of the hydrocarbon system. The retrograde liquid in the reservoir at any time is composed of mostly methane and ethane by volume, and so it is much larger than the volume of stable liquid that could be obtained from it at atmospheric temperature and pressure. The composition of this retrograde liquid is changing as pressure declines so that 4% retrograde liquid volume at, for example, 750 psia might contain as much surface condensate as 6% retrograde liquid volume at 2250 psia.
If the initial reservoir fluid composition is found at 2900 psia and 75°F, point C, the reservoir would be in a one-phase state, now called liquid, because the temperature is below the critical-point temperature. This is called a bubble-point (or black-oil or solution-gas) reservoir. As pressure declines during production, the bubble-point pressure will be reached, in this case at 2550 psia, point C1. Below this pressure, bubbles, or a free-gas phase, will appear. When the free gas saturation is sufficiently large, gas flows to the wellbore in ever increasing quantities. Because surface facilities limit the gas production rate, the oil flow rate declines, and when the oil rate is no longer economic, much unrecovered oil remains in the reservoir.
Finally, if the initial hydrocarbon mixture occurred at 2000 psia and 150°F, point D, it would be a two-phase reservoir, consisting of a liquid or oil zone overlain by a gas zone or cap. Because the composition of the gas and oil zones are entirely different from each other, they may be represented separately by individual phase diagrams that bear little relation to each other or to the composite. The liquid or oil zone will be at its bubble point and will be produced as a bubble-point reservoir modified by the presence of the gas cap. The gas cap will be at the dew point and may be either retrograde, as shown in Fig. 1.5(a), or nonretrograde, as shown in Fig. 1.5(b).

Figure 1.5 Phase diagrams of a cap gas and oil zone fluid showing (a) retrograde cap gas and (b) nonretrograde cap gas.
From this technical point of view, hydrocarbon reservoirs are initially either in a single-phase state (A, B, or C) or in a two-phase state (D), depending on their temperatures and pressures relative to their phase envelopes. Table 1.2 depicts a summary of these four types. These reservoir types are discussed in detail in Chapters 4, 5, 6, and 7, respectively.
Table 1.2 Summary of Reservoir Types
Type A single phase gas |
Type B gas condensate |
Type C undersaturated oil |
Type D saturated oil |
|
Typical primary recovery mechanism |
Volumetric gas drive |
Volumetric gas drive |
Depletion drive, water drive |
Volumetric gas drive, depletion drive, water drive |
Initial reservoir conditions |
Single phase: Gas |
Single phase: Gas |
Single phase: Oil |
Two phase: Oil and gas |
Reservoir behavior as pressure declines |
Reservoir fluid remains as gas. |
Liquid condenses in the reservoir. |
Gas vaporizes in reservoir. |
Saturated oil releases additional gas. |
Produced hydrocarbons |
Primarily gas |
Gas and condensate |
Oil and gas |
Oil and gas |
Table 1.3 presents the mole compositions and some additional properties of five single-phase reservoir fluids. The volatile oil is intermediate between the gas condensate and the black, or heavy, oil types. Production with gas-oil ratios greater than 100,000 SCF/STB is commonly called lean or dry gas, although there is no generally recognized dividing line between the two categories. In some legal work, statutory gas wells are those with gas-oil ratios in excess of 100,000 SCF/STB. The term wet gas is sometimes used interchangeably with gas condensate. In the gas-oil ratios, general trends are noticeable in the methane and heptanes-plus content of the fluids and the color of the tank liquids. Although there is good correlation between the molecular weight of the heptanes plus and the gravity of the stock-tank liquid, there is virtually no correlation between the gas-oil ratios and the gravities of the stock-tank liquids, except that most black oil reservoirs have gas-oil ratios below 1000 SCF/STB and stock-tank liquid gravities below 45 °API. The gas-oil ratios are a good indication of the overall composition of the fluid, high gas-oil ratios being associated with low concentrations of pentanes and heavier and vice versa.
Table 1.3 Mole Composition and Other Properties of Typical Single-Phase Reservoir Fluids
Component |
Black oil |
Volatile oil |
Gas condensate |
Dry gas |
Wet gas |
C1 |
48.83 |
64.36 |
87.07 |
95.85 |
86.67 |
C2 |
2.75 |
7.52 |
4.39 |
2.67 |
7.77 |
C3 |
1.93 |
4.74 |
2.29 |
0.34 |
2.95 |
C4 |
1.60 |
4.12 |
1.74 |
0.52 |
1.73 |
C5 |
1.15 |
2.97 |
0.83 |
0.08 |
0.88 |
C6 |
1.59 |
1.38 |
0.60 |
0.12 |
|
42.15 |
14.91 |
3.80 |
0.42 |
||
Total |
100.00 |
100.00 |
100.00 |
100.00 |
100.00 |
Mol. wt. |
225 |
181 |
112 |
157 |
|
GOR, SCF/STB |
625 |
2000 |
18,200 |
105,000 |
Infinite |
Tank gravity, °API |
34.3 |
50.1 |
60.8 |
54.7 |
|
Liquid color |
Greenish black |
Medium orange |
Light straw |
Water white |
The gas-oil ratios given in Table 1.3 are for the initial production of the one-phase reservoir fluids producing through one or more surface separators operating at various temperatures and pressures, which may vary considerably among the several types of production. The gas-oil ratios and consequently the API gravity of the produced liquid vary with the number, pressures, and temperatures of the separators so that one operator may report a somewhat different gas-oil ratio from another, although both produce the same reservoir fluid. Also, as pressure declines in the black oil, volatile oil, and some gas-condensate reservoirs, there is generally a considerable increase in the gas-oil ratio owing to the reservoir mechanisms that control the relative flow of oil and gas to the wellbores. The separator efficiencies also generally decline as flowing wellhead pressures decline, which also contributes to increased gas-oil ratios.
What has been said previously applies to reservoirs initially in a single phase. The initial gas-oil ratio of production from wells completed either in the gas cap or in the oil zone of two-phase reservoirs depends, as discussed previously, on the compositions of the gas cap hydrocarbons and the oil zone hydrocarbons, as well as the reservoir temperature and pressure. The gas cap may contain gas condensate or dry gas, whereas the oil zone may contain black oil or volatile oil. Naturally, if a well is completed in both the gas and oil zones, the production will be a mixture of the two. Sometimes this is unavoidable, as when the gas and oil zones (columns) are only a few feet in thickness. Even when a well is completed in the oil zone only, the downward coning of gas from the overlying gas cap may occur to increase the gas-oil ratio of the production.