- 1.1 The Structure of Complex Systems
- 1.2 The Inherent Complexity of Software
- 1.3 The Five Attributes of a Complex System
- 1.4 Organized and Disorganized Complexity
- 1.5 Bringing Order to Chaos
- 1.6 On Designing Complex Systems
- Summary
1.4 Organized and Disorganized Complexity
The discovery of common abstractions and mechanisms greatly facilitates our understanding of complex systems. For example, with just a few minutes of orientation, an experienced pilot can step into a multiengine jet aircraft he or she has never flown before and safely fly the vehicle. Having recognized the properties common to all such aircraft, such as the functioning of the rudder, ailerons, and throttle, the pilot primarily needs to learn what properties are unique to that particular aircraft. If the pilot already knows how to fly a given aircraft, it is far easier to learn how to fly a similar one.
The Canonical Form of a Complex System
This example suggests that we have been using the term hierarchy in a rather loose fashion. Most interesting systems do not embody a single hierarchy; instead, we find that many different hierarchies are usually present within the same complex system. For example, an aircraft may be studied by decomposing it into its propulsion system, flight-control system, and so on. This decomposition represents a structural, or "part of" hierarchy.
Alternately, we can cut across the system in an entirely orthogonal way. For example, a turbofan engine is a specific kind of jet engine, and a Pratt and Whitney TF30 is a specific kind of turbofan engine. Stated another way, a jet engine represents a generalization of the properties common to every kind of jet engine; a turbofan engine is simply a specialized kind of jet engine, with properties that distinguish it, for example, from ramjet engines.
This second hierarchy represents an "is a" hierarchy. In our experience, we have found it essential to view a system from both perspectives, studying its "is a" hierarchy as well as its "part of" hierarchy. For reasons that will become clear in the next chapter, we call these hierarchies the class structure and the object structure of the system, respectively.2
For those of you who are familiar with object technology, let us be clear. In this case, where we are speaking of class structure and object structure, we are not referring to the classes and objects you create when coding your software. We are referring to classes and objects, at a higher level of abstraction, that make up complex systems, for example, a jet engine, an airframe, the various types of seats, an autopilot subsystem, and so forth. You will recall from the earlier discussion on the attributes of a complex system that whatever is considered primitive is relative to the observer.
In Figure 1-1 we see the two orthogonal hierarchies of the system: its class structure and its object structure. Each hierarchy is layered, with the more abstract classes and objects built on more primitive ones. What class or object is chosen as primitive is relative to the problem at hand. Looking inside any given level reveals yet another level of complexity. Especially among the parts of the object structure, there are close collaborations among objects at the same level of abstraction.
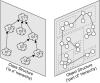
Figure 1-1 The Key Hierarchies of Complex Systems
Combining the concept of the class and object structures together with the five attributes of a complex system (hierarchy, relative primitives [i.e., multiple levels of abstraction], separation of concerns, patterns, and stable intermediate forms), we find that virtually all complex systems take on the same (canonical) form, as we show in Figure 1-2. Collectively, we speak of the class and object structures of a system as its architecture.
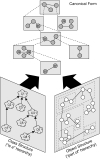
Figure 1-2 The Canonical Form of a Complex System
Notice also that the class structure and the object structure are not completely independent; rather, each object in the object structure represents a specific instance of some class. (In Figure 1-2, note classes C3, C5, C7, and C8 and the number of the instances 03, 05, 07, and 08.) As the figure suggests, there are usually many more objects than classes of objects within a complex system. By showing the "part of" as well as the "is a" hierarchy, we explicitly expose the redundancy of the system under consideration. If we did not reveal a system's class structure, we would have to duplicate our knowledge about the properties of each individual part. With the inclusion of the class structure, we capture these common properties in one place.
Also from the same class structure, there are many different ways that these objects can be instantiated and organized. No one particular architecture can really be deemed "correct." This is what makes system architecture challenging—finding the balance between the many ways the components of a system can be structured, the five attributes of complex systems, and the needs of the system user.
Our experience is that the most successful complex software systems are those whose designs explicitly encompass well-engineered class and object structures and embody the five attributes of complex systems described in the previous section. Lest the importance of this observation be missed, let us be even more direct: We very rarely encounter software systems that are delivered on time, that are within budget, and that meet their requirements, unless they are designed with these factors in mind.
The Limitations of the Human Capacity for Dealing with Complexity
If we know what the design of complex software systems should be like, then why do we still have serious problems in successfully developing them? This concept of the organized complexity of software (whose guiding principles we call the object model) is relatively new. However, there is yet another factor that dominates: the fundamental limitations of the human capacity for dealing with complexity.
As we first begin to analyze a complex software system, we find many parts that must interact in a multitude of intricate ways, with little perceptible commonality among either the parts or their interactions; this is an example of disorganized complexity. As we work to bring organization to this complexity through the process of design, we must think about many things at once. For example, in an air traffic control system, we must deal with the state of many different aircraft at once, involving such properties as their location, speed, and heading. Especially in the case of discrete systems, we must cope with a fairly large, intricate, and sometimes nondeterministic state space. Unfortunately, it is absolutely impossible for a single person to keep track of all of these details at once. Experiments by psychologists, such as those of Miller, suggest that the maximum number of chunks of information that an individual can simultaneously comprehend is on the order of seven, plus or minus two [14]. This channel capacity seems to be related to the capacity of short-term memory. Simon additionally notes that processing speed is a limiting factor: It takes the mind about five seconds to accept a new chunk of information [15].
We are thus faced with a fundamental dilemma. The complexity of the software systems we are asked to develop is increasing, yet there are basic limits on our ability to cope with this complexity. How then do we resolve this predicament?